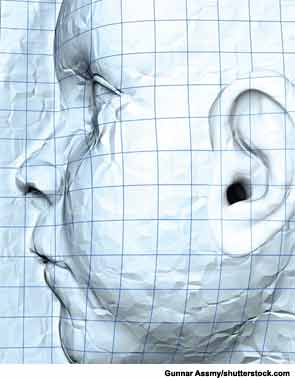
Kaiba Gionfriddo, after several episodes of airway obstruction, was diagnosed with tracheobronchomalacia, with a compressed left mainstem bronchus and transposed pulmonary arteries. Kaiba had undergone tracheostomy and been placed on a ventilator. But, said Glenn Green, MD, associate professor of otolaryngology at the University of Michigan Medical School, Ann Arbor, the episodes continued.
Explore This Issue
July 2013So Dr. Green and his colleagues printed out an airway stent for him.
They used a technology popularly known as “3D printing,” but the more formal and perhaps more descriptive term is “additive manufacturing,” because the process creates products through the accumulation of many thin layers of material. Additive manufacturing devices have been around for decades, but, until recently, their size, complexity and questionable reliability made them impractical for routine use.
Within the past few years, however, they have become faster, more consistent, easier to use and affordable: Some desktop models are available for as little as $300. Current 3D printers can quickly produce items of Byzantine intricacy that might be impossible to create in any other way, ranging from tiny nuts and bolts to large architectural structures. They can make household items such as lamps or pens, clothing such as shoes and, in one notorious example, a gun.
“The printer obtains the information in basically the same way as when you send a Word document to your printer, and it takes those data and prints them out,” said Jason A. Spector, MD, associate professor of surgery at New York-Presbyterian/Weill Cornell Medical Center in New York City. For a 3D printer, the data in question come as three-dimensional geometric renderings of the desired item, which are developed through computer-aided design. Using those renderings as a template, the printer then builds the item layer by micron-thin layer, using the material of which the item is made as the “ink.” These printers lend themselves to medical applications because they can rapidly and efficiently custom tailor devices for specific patients from MRI or CT images, and they can create porous, honeycombed structures for implants such as knee joints. Various laboratories have even “printed” prototypes of lungs, teeth and kidneys, as well as ears—and airway stents.
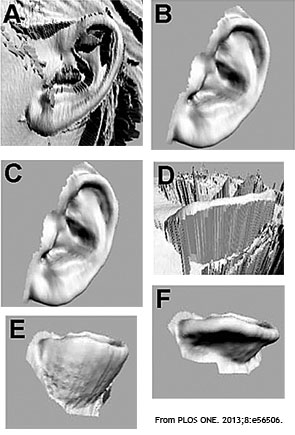
Opening an Airway
Dr. Green and his colleague Scott Hollister, PhD, professor of biomedical engineering, had already been exploring ways to make implants using the resorbable polymer polycaprolactone. For Kaiba, they obtained emergency clearance from the Food and Drug Administration to use the material to make a tracheal stent.
Armed with CT images of the child’s airway, they fashioned the stent according to a bellowed typology design, so that it resembled a tiny vacuum cleaner hose, flexible but sturdy (see image, right) Unlike stem cell-derived tracheas that have also been in the news, “this design specifically does not collapse,” said Dr. Green. “This enhances its applicability. The stent puts the patient’s natural trachea into the correct position, rather than replacing the patient’s tissues with tissue derived from stem cells.” The stent was placed around the collapsed portion of Kaiba’s left bronchus and sutured in place. Although the surgery occurred in February 2012, it was not announced to the general public until last month.
The procedure was not without its risks. Kaiba had multiple comorbidities and “was not the perfect candidate,” said Dr. Green. “But his life was dependent upon it. He was still coding, even with a tracheostomy and mechanical ventilation. It was just a matter of weeks.” Today, he is doing “fabulously,” he said. “He’s had no episodes of cyanosis since the surgery, and he has a normal life expectancy.”

Creating an Ear
Dr. Spector, who specializes in plastic and reconstructive surgery, and his colleagues are using 3D printing to make external ears that capture the biomechanical properties of normal human ears. Their goal is to provide a cosmetically and physiologically sound option for children with microtia.
They use a special 3D camera to gather the original source data, with the child’s opposite ear as a model. This step takes about 30 seconds. “Once the data are obtained and put into the work station, they are manipulated until we have a three-dimensional or digitized rendering of the ear. We then use that information to build the actual 3D replica of the ear,” Dr. Spector said. Their “ink” is a collagen hydrogel seeded with bovine auricular chondrocytes (see images).
This work is still experimental, but in a recent study they compared the cellular prototypes with ears developed from the collagen hydrogel alone, with no added chondrocytes (PLOS ONE. 2013;8:e56506). Ears of each type were implanted subcutaneously on the backs of nude rats. Within one month, the acellular constructs had deteriorated significantly compared with the cell-seeded ears, which retained their size and shape. The differences were even more striking at three months, and the cell-seeded ears had the same biomechanical properties as native auricular cartilage (see image, left).
One of the greatest challenges to clinical use lies in finding a plentiful, reliable source of chondrocytes, Dr. Spector said. He estimates that it will require 250 million cells to properly build an ear. “We’re working on a way of expanding the small number of cartilage cells derived from patient biopsies by mixing them with stem cells or other growth factors to turbo-charge their rates of reproduction.”
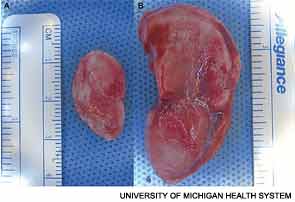
The Bionic Ear
Another team of researchers, led by Michael McAlpine, PhD, assistant professor of mechanical and aerospace engineering at Princeton University, has fused biology with electronics to create a structure that not only looks like a human ear but also contains a tiny, coiled antenna that can pick up radiofrequency signals. Like Dr. Spector and his colleagues, Dr. McAlpine and colleagues used calf chondrocytes to make the ear itself. For the antenna, they added a polymer infused with silver nanoparticles. “We used a computer software program that defines the structure in three dimensions and also defines where each material should be placed in a layer-by-layer process,” Dr. McAlpine said. “We then fed nanoelectronics, biological and structural components into the printer.” The result: an ear that can receive signals beyond normal human range.
Their goal was to demonstrate the feasibility of merging electronic and biological systems, said David H. Gracias, PhD, associate professor of chemical and biomolecular engineering at Johns Hopkins University in Baltimore, who collaborated with Dr. McAlpine on this research. “Tissues typically are soft and full of water, and there’s a fundamental mismatch between them and electronics, which are made of metal and are fabricated in a dry vacuum and do not like water. Moreover, it’s a challenge to bridge the interface, because metals don’t adhere well to polymers and biological substrates. This research was a proof-of-concept demonstration of the idea that by printing and embedding the antenna within the tissue structure, it was possible to bridge this divide.”
Dr. Gracias can’t help expressing a note of wonderment at these developments. “It’s quite amazing that you can actually print the cells, that they live, they appear to have the morphology of the ear and the antenna is printed and can receive a signal. The idea that you can keep something alive and fuse it with an electronic device and actually get it to work—that’s really something.”