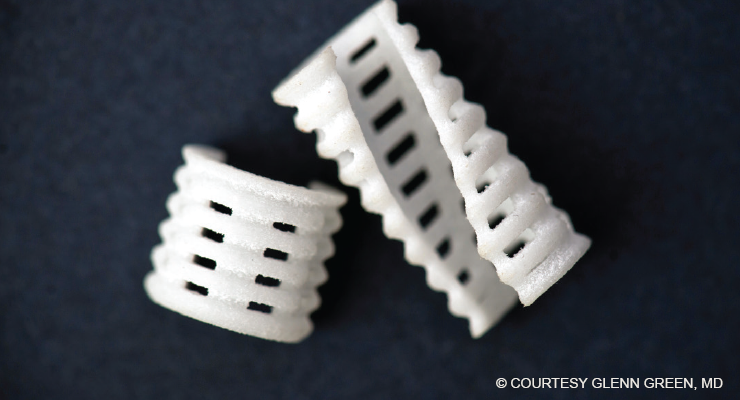
Fig. 1. A resorbable trachea stent used in the treatment of tracheobronchomalacia in infants.
Three-dimensional (3D) printing is transforming the field of pediatric otolaryngology. Nine years after implanting the first 3D bioabsorbable tracheal splint in an infant boy with severe tracheobronchomalacia (N Engl J Med. 2013;368:2043-2045), a multidisciplinary team of surgeons and biomedical engineers has successfully created a neotrachea supported by a 3D-printed scaffold to treat an infant born with tracheal agenesis, a rare congenital anomaly that’s usually fatal.
Explore This Issue
February 2021“3D printing is allowing us to treat diseases that weren’t treatable before,” said Glenn Green, MD, professor of otolaryngology–head and neck surgery at the University of Michigan Medical School, Ann Arbor, and one of the lead surgeons involved in both cases. “It’s opening up new ways of making devices that are specific for individuals who have rare conditions who, if left untreated, would not survive.” The infant was the first child to undergo this procedure; she’s doing well and is already the second-longest surviving child born with this condition in the United States, Dr. Green noted.
Tracheal Stent
Although there are many surgical strategies for managing tracheobronchomalacia, such as tracheostomy, tracheal resection, and slide tracheoplasty, before the advent of the 3D approach, surgeons were running out of options for treating very ill infants. The idea for creating a 3D trachea stent was born out of a conversation Dr. Green had with a colleague. “I was talking about how we had these kids whom we were unable to treat. I was introduced to Scott Hollister, PhD, an engineer, and together we developed something that has helped a lot of children.” Dr. Hollister is now a bioengineer in the Wallace H. Coulter Department of Biomedical Engineering at the Georgia Institute of Technology in Atlanta.
First implanted in 2012, the 3D-printed scaffold stent is designed to go around the outside of the diseased portion of the trachea to allow air to freely flow into the lungs (Fig. 1). To date, Dr. Green and his colleagues at the University of Michigan have treated 34 patients using the device. “We’ve taken individuals who were unable to leave the intensive care unit for months due to serious episodes of respiratory obstruction, and they’re now home with their parents. These conditions are now very treatable,” Dr. Green said.
Because the implant is porous, it creates a conductive space for blood vessels to grow. Within the first couple of weeks, the patient’s own blood vessels quickly grow into the scaffolding. —David A. Zopf, MD, MS
One of the main benefits of the device is that it’s made of a resorbable material designed to dissolve after approximately three to four years. As the tissue grows into the stent, it’s reconfigured to the shape of the scaffold. “By reconfiguring the way that the tissue grows, over time the original problem goes away,” said Dr. Green. “We now have about nine years of follow-up, and our early patients are growing and thriving.”
Dr. Green and his University of Michigan colleagues are in the process of applying for FDA approval for the splint procedure so that it can be made available to a wider group of surgeons and patients.
Multiple Benefits of 3D Printing
The application of 3D printing in otolaryngology falls into four broad categories: presurgical models, educational models for training, medical devices, and tissue engineering, according to Robert J. Morrison, MD, assistant professor in the department of otolaryngology–head and neck surgery at the University of Michigan. Early on, 3D printers were used to construct surgical models for preoperative planning and intraoperative guides; these models can also be used as educational models for surgical simulation (see “3D Simulators as Training Tools” on page 12).
None of these intricate surgeries would be possible without the field of tissue engineering. Tissue engineering falls into two categories, Dr. Morrison noted: scaffold-based and bioprinting. In the scaffold-based approach, cells are seeded into the scaffolding. As the scaffold gradually dissolves, “you’re left with a structure that’s made out of that native tissue in the desired 3D shape that you want,” Dr. Morrison said.
The advantage of 3D printing is the ability to customize the stent for each individual patient and avoid many of the pitfalls of traditional metal and silicone stents. “3D printing has become nearly synonymous with patient-specific manufacturing,” said Joshua A. Stramiello, MD, a resident in the division of otolaryngology–head and neck surgery, department of surgery, University of California, San Diego.
In a review of 3D-printed stent procedures in children, Dr. Stramiello and his colleagues found that, “given recent improvements in materials science, there is a growing body of evidence suggesting a strong role for bioresorbable intraluminal stents in treating pediatric tracheobronchial obstruction” (Int J Pediatr Otorhinolaryngol. 2020;132:109923).
These procedures aren’t without risks, however. In a pooled analysis of pediatric trials of scaffold-based procedures using intraluminal polydioxanone (PDO) stents, Dr. Stramiello and his colleagues reported an overall complication rate of 21.7%, with a stent fragment foreign body being the most common (8.7%), followed by significant granulation tissue (4.3%), stent migration (4.3%), and local stenosis (4.3%) (Int J Pediatr Otorhinolaryngol. 2020;139:110405). Dr. Stramiello noted that although comparative animal studies report biocompatibility and fewer morbidities compared with metal and silicone stents, in human studies, there are concerns over the short interval of degradation and the potential for obstructive foreign bodies in poorly seated stents.
[3D printing is] opening up new ways of making devices that are specific for individuals who have rare conditions who, if left untreated, would not survive. —Glenn Green, MD
“Fortunately, the treatment success using the 3D-printed airway device [polycaprolactone] has just been miraculous,” said David A. Zopf, MD, MS, assistant professor in the department of otolaryngology–head and neck surgery, University of Michigan, who was part of the team that conducted the first tracheal splint procedure. “Despite this being used in critically ill patients who have not responded to standard treatments, the number of complications has been exceedingly rare,” he said (Laryngoscope. 2019;129:1763-1771).
Bioprinting
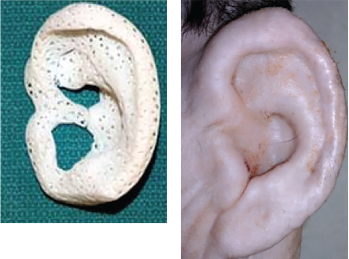
© David A. Zopf, MD, MS
Fig. 2. Images of a 3D-printed ear, and the ear after implantation.
Bioprinting, a procedure in which a 3D printer actually prints out the cells embedded in a hydrogel, has been much more challenging to perfect, Dr. Morrison said.
“The challenge with tracheal tissue engineering is reproducing the mucosa. If we don’t recreate the ciliated lining, there’s a risk of crusting, mucus buildup, and airway obstruction,” Dr. Morrison explained.
Bioprinting is still experimental. Although scientists have had good success growing cell cultures in the laboratory, the yield “tends to be thin layers of cells,” Dr. Morrison said. When it comes to tissue engineering, the biggest challenge is to create something that’s thicker than just a layer of cells on a Petri dish. “You need to be able to generate a robust blood supply to support thicker bone or cartilage 3D structures,” he said. To that end, he noted, researchers have begun to investigate bioprinting vascular cells and channels into their tissue-engineered structure.
One researcher who’s working hard to break the code is Lee P. Smith, MD, chief of the division of pediatric otolaryngology, Steven and Alexandra Cohen Children’s Medical Center, New Hyde Park, Hofstra North Shore-LIJ School of Medicine in Hempstead, N.Y. In 2015, Dr. Smith and his colleagues created a tissue-engineered, biodegradable 3D-printed tracheal ring as a first step toward bioengineering a long-segment tracheal replacement for patients with tracheomalacia and stenosis. The cells in the scaffold proliferated and maintained their cartilage phenotype without altering the mechanical properties of the scaffold, Dr. Smith wrote in the study describing the technique (Open J Regen Med. 2015;4:27-33).
Dr. Smith and his colleagues have also combined 3D printing and tissue engineering to produce a living biological graft for laryngotracheal reconstruction (LTR). As a first step, the team created a 3D computer model of an anterior LTR graft and then printed a laryngotracheal scaffold with polylactic acid. The scaffolds were seeded with mature chondrocytes and collagen gel and implanted in nine New Zealand white rabbits. According to Dr. Smith, the in vitro cell proliferation assay demonstrated an initial viability of 87.5% and the cells doubled over the first seven days (Otolaryngol Head Neck Surg. 2015;153:1001-1006). “The cartilage in our graft maintained its functional characteristics and all of the animals survived the study period without respiratory distress,” he said. More recent studies have evaluated the potential to use this procedure in a segmental tracheal defect (Int J Ped Oto. 2019;117:175-178)
“Our results indicate that it’s possible to produce a custom-designed, 3D-printed, tissue-engineered graft for airway reconstruction,” Dr. Smith noted. However, he cautioned that “we are at least five to seven years away from being able to apply these techniques to humans.”
“I think [advances in tracheal tissue engineering] will require some combination of these approaches—scaffolding and bioprinting—that ultimately cracks the code and gives us something that we can use in humans,” said Dr. Morrison.
3D Applications Beyond the Trachea
One of the major benefits of 3D printers has been the ability to custom-make a patient-specific device from an MRI or CT image. This has enabled surgeons to recreate an ear or a nose or to manufacture custom-made tracheal plugs.
“What our group has been able to do is take a broad look at a problem and make advancements that are meaningful for patients,” Dr. Zopf said. One example of this has been his work on creating an outer ear for children born with microtia (congenital absence of the ear). Using the same material and scaffold-based technique developed for the tracheal stents, Dr. Zopf has been able to create ear soft tissue foundation that has the same architecture as cartilage (Fig. 2).
“Traditionally, we construct the framework of the ear from the ribs of the children [at age eight to nine years] in the operating room. With 3D printing, we could scan the patient’s normal ear and create a patient-specific implant that’s a more precise model than we could have created by hand.” Dr. Zopf and his colleagues have piloted this procedure in a large animal model (Laryngoscope. Published online October 6, 2020).
When implanted, bioengineered cartilage expanded from conchal cartilage into the scaffolding could over time replace the resorbable scaffold with the patient’s own tissue. “Because the implant is porous [printed in bioresorbable polycaprolactone with orthogonally interconnected spherical pores], it creates a conductive space for blood vessels to grow. Within the first couple of weeks, the patient’s own blood vessels quickly grow into the scaffolding—which has previously been a huge obstacle in tissue engineering. We are not tissue-engineering the blood vessels, but just encouraging the patient’s own blood vessels to join the party,” Dr. Zopf said.
Because these children are born with cochlea and the cochlear nerve, Dr. Zopf’s team can also restore hearing on the affected side by implanting a bone conduction device at the time of the reconstruction.
3D printing also has a wide application for cancer and trauma patients. “From our experience with the airway stent, we now know how to create 3D-printed noses using the same scaffold-based technique,” noted Dr. Zopf. “This has created enormous potential for cancer and trauma patients.”
Nikki Kean is a freelance medical writer based in New Jersey.
3D Simulators as Training Tools
Because of the COVID-19 pandemic and social distancing, much of the hands-on cadaver and animal model training has halted. This pause has opened the door to a more cost-effective yet still hands-on experience—3D models.
The American Society of Pediatric Otolaryngology has used 3D printing techniques to conduct a fellows simulator day for chief residents who have matched into a pediatric otolaryngology fellowship. “We have developed a number of simulators now for procedures that would fall under the umbrella of complex pediatric ENT surgeries,” said Robert J. Morrison, MD, assistant professor in the department of otolaryngology–head and neck surgery at the University of Michigan. “This is a chance for the incoming fellows to develop some familiarity with the technique of those operations prior to starting their fellowship, which is unbelievable.”
“We have found that 3D printing for surgical planning not only improves preoperative assessment of surgical approach and stent customization, but also provides an excellent model for educating parents and patients about the condition,” said Joshua A. Stramiello, MD, a resident in the division of otolaryngology–head and neck surgery, department of surgery, University of California, San Diego.
These 3D-printed simulators offer another benefit: the ability to train surgeons in countries where there’s a shortage of trained healthcare professionals. Dr. Zopf and colleagues conducted a study of 3D-printed simulators in Ethiopia, where the model is used to teach clinicians how to perform emergency airway surgery (Int J Ped Otolaryngol. 2018;114:124-128).
“We’re training local surgeons first on a high-fidelity simulator prior to exposure to patients so that they can then perform the procedures once the team leaves. We’re teaching them to fish for themselves, so to speak, to provide a sustainable solution for regions in need,” said David A. Zopf, MD, MS, assistant professor in the department of otolaryngology–head and neck surgery, University of Michigan, referring to the oft-quoted adage on self-sufficiency.