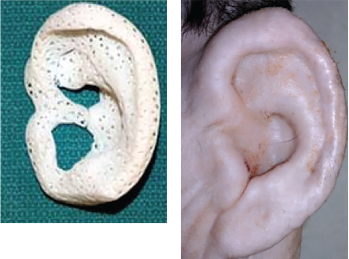
© David A. Zopf, MD, MS
Explore This Issue
February 2021Fig. 2. Images of a 3D-printed ear, and the ear after implantation.
Bioprinting, a procedure in which a 3D printer actually prints out the cells embedded in a hydrogel, has been much more challenging to perfect, Dr. Morrison said.
“The challenge with tracheal tissue engineering is reproducing the mucosa. If we don’t recreate the ciliated lining, there’s a risk of crusting, mucus buildup, and airway obstruction,” Dr. Morrison explained.
Bioprinting is still experimental. Although scientists have had good success growing cell cultures in the laboratory, the yield “tends to be thin layers of cells,” Dr. Morrison said. When it comes to tissue engineering, the biggest challenge is to create something that’s thicker than just a layer of cells on a Petri dish. “You need to be able to generate a robust blood supply to support thicker bone or cartilage 3D structures,” he said. To that end, he noted, researchers have begun to investigate bioprinting vascular cells and channels into their tissue-engineered structure.
One researcher who’s working hard to break the code is Lee P. Smith, MD, chief of the division of pediatric otolaryngology, Steven and Alexandra Cohen Children’s Medical Center, New Hyde Park, Hofstra North Shore-LIJ School of Medicine in Hempstead, N.Y. In 2015, Dr. Smith and his colleagues created a tissue-engineered, biodegradable 3D-printed tracheal ring as a first step toward bioengineering a long-segment tracheal replacement for patients with tracheomalacia and stenosis. The cells in the scaffold proliferated and maintained their cartilage phenotype without altering the mechanical properties of the scaffold, Dr. Smith wrote in the study describing the technique (Open J Regen Med. 2015;4:27-33).
Dr. Smith and his colleagues have also combined 3D printing and tissue engineering to produce a living biological graft for laryngotracheal reconstruction (LTR). As a first step, the team created a 3D computer model of an anterior LTR graft and then printed a laryngotracheal scaffold with polylactic acid. The scaffolds were seeded with mature chondrocytes and collagen gel and implanted in nine New Zealand white rabbits. According to Dr. Smith, the in vitro cell proliferation assay demonstrated an initial viability of 87.5% and the cells doubled over the first seven days (Otolaryngol Head Neck Surg. 2015;153:1001-1006). “The cartilage in our graft maintained its functional characteristics and all of the animals survived the study period without respiratory distress,” he said. More recent studies have evaluated the potential to use this procedure in a segmental tracheal defect (Int J Ped Oto. 2019;117:175-178)
“Our results indicate that it’s possible to produce a custom-designed, 3D-printed, tissue-engineered graft for airway reconstruction,” Dr. Smith noted. However, he cautioned that “we are at least five to seven years away from being able to apply these techniques to humans.”
“I think [advances in tracheal tissue engineering] will require some combination of these approaches—scaffolding and bioprinting—that ultimately cracks the code and gives us something that we can use in humans,” said Dr. Morrison.
3D Applications Beyond the Trachea
One of the major benefits of 3D printers has been the ability to custom-make a patient-specific device from an MRI or CT image. This has enabled surgeons to recreate an ear or a nose or to manufacture custom-made tracheal plugs.
“What our group has been able to do is take a broad look at a problem and make advancements that are meaningful for patients,” Dr. Zopf said. One example of this has been his work on creating an outer ear for children born with microtia (congenital absence of the ear). Using the same material and scaffold-based technique developed for the tracheal stents, Dr. Zopf has been able to create ear soft tissue foundation that has the same architecture as cartilage (Fig. 2).
“Traditionally, we construct the framework of the ear from the ribs of the children [at age eight to nine years] in the operating room. With 3D printing, we could scan the patient’s normal ear and create a patient-specific implant that’s a more precise model than we could have created by hand.” Dr. Zopf and his colleagues have piloted this procedure in a large animal model (Laryngoscope. Published online October 6, 2020).
When implanted, bioengineered cartilage expanded from conchal cartilage into the scaffolding could over time replace the resorbable scaffold with the patient’s own tissue. “Because the implant is porous [printed in bioresorbable polycaprolactone with orthogonally interconnected spherical pores], it creates a conductive space for blood vessels to grow. Within the first couple of weeks, the patient’s own blood vessels quickly grow into the scaffolding—which has previously been a huge obstacle in tissue engineering. We are not tissue-engineering the blood vessels, but just encouraging the patient’s own blood vessels to join the party,” Dr. Zopf said.
Because these children are born with cochlea and the cochlear nerve, Dr. Zopf’s team can also restore hearing on the affected side by implanting a bone conduction device at the time of the reconstruction.
3D printing also has a wide application for cancer and trauma patients. “From our experience with the airway stent, we now know how to create 3D-printed noses using the same scaffold-based technique,” noted Dr. Zopf. “This has created enormous potential for cancer and trauma patients.”
Nikki Kean is a freelance medical writer based in New Jersey.